Translate this page into:
Diagnostic Utility of Urine Microscopy in Kidney Diseases
Corrresponding author: Payal Gaggar, Department of Nephrology, Medical Trust Hospital, Kochi, Kerala, India. E-mail: payalgaggar04@gmail.com
-
Received: ,
Accepted: ,
How to cite this article: Gaggar P, Raju SB. Diagnostic Utility of Urine Microscopy in Kidney Diseases. Indian J Nephrol. 2024;34:213–21. doi: 10.25259/ijn_362_23.
Abstract
Urine sediment analysis is a highly valuable yet underutilized test in today’s advanced medical landscape. The analysis of urine sediment is a simple, cost-effective, and powerful diagnostic tool in the hands of a skilled nephrologist, generally in all kidney diseases and particularly more so in the setting of acute kidney injury (AKI). The impact of AKI is far-reaching and encompasses elevated mortality rates, increased morbidity, longer hospital stays, and higher overall healthcare expenses. Timely and compartmental diagnosis of AKI with the use of a simple urine sediment analysis leads to targeted therapeutic strategies and also serves as a prognostic guide. The widespread use of automated analysis in recent times has its own set of limitations, as it fails to identify pathological casts, crystals, and dysmorphic red blood cells (RBCs). Hence, it is the need of the hour to learn this time-honored art of urine sediment analysis, to provide comprehensive patient care.
Keywords
AKI
kidney diseases
microscopy
urine sediment
Introduction
Rayer and his students in Paris pioneered clinical urine microscopy in the late 1830s. As microscope technology advanced in the mid-1900s, urine sediment analysis became an essential component of clinical examination.1 Careful analysis of the concentrated particles in urine under a microscope, performed by an experienced nephrologist, plays a vital role in both the identification and treatment of various kidney-related ailments. A skillful and motivated urine sediment examination provides a window into the kidney, and hence has been considered as the “liquid biopsy.” Utilizing it as a diagnostic aid proves particularly beneficial when evaluating individuals with kidney diseases in general and acute kidney injury (AKI) in particular. Acute tubular necrosis (ATN) is the leading cause of AKI in hospitalized patients, followed by prerenal azotemia.2 Since the treatment approaches and outlook for prerenal azotemia and ATN significantly vary, it is crucial to distinguish them promptly based on clinical indicators and laboratory parameters.
In this review, we will discuss the various components of urine sediment [Figure 1] seen in the different etiologies of AKI – prerenal AKI, ATN, acute interstitial nephritis (AIN), nephrotic syndrome, nephritic syndrome, and crystal-induced AKI.
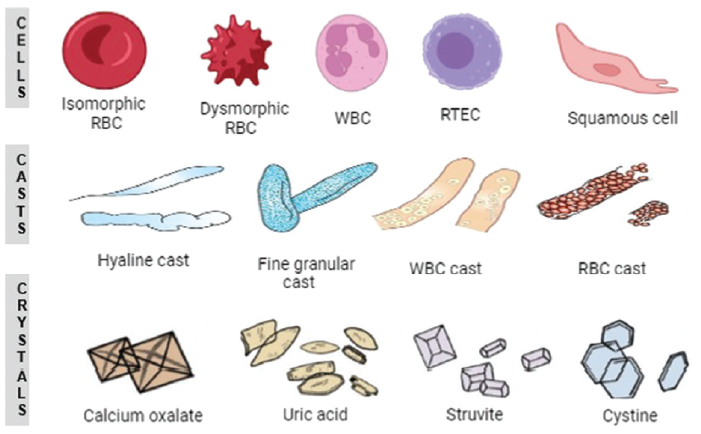
- Different types of formed elements seen in the urine sediment analysis. RBC: red blood cell, WBC: white blood cell, RTEC: Renal tubular epithelial cells.
Procedure of urine sediment analysis
For sediment analysis, a second catch sample is preferred. The first void sample is susceptible to lysis of cells and casts [Tables 1 and 2] after prolonged standing of urine in the bladder overnight, hence should be avoided. An alkaline pH and a low osmolality of urine lead to lysis of cells and casts, making interpretation of urine sediment difficult. To prevent material breakdown, the European analysis guidelines recommend examining urinary particles within 1 h after voiding at room temperature or within 4 h if refrigerated. To ensure accurate identification of crystals, a separate nonrefrigerated aliquot is essential, as refrigeration can cause phosphates and urates to precipitate. If the urine sample cannot be analyzed within 4 h of refrigeration, preservatives such as formaldehyde-based solutions, buffered boric acid, and formate-based solutions should be added. However, the presence of preservatives can potentially impact the chemical characteristics and change the appearance of particles. Therefore, it is recommended to analyze the sample promptly after voiding.3 About 10 ml of fresh/properly stored urine is centrifuged in a conical tube at 400 g for at least 5 min. By utilizing suction, a quantity of 9.5 ml of supernatant urine is extracted, leaving behind the pellet. The pellet is suspended in the small amount of urine that remains in the tube through gentle agitation. A standardized glass slide is employed, and using a pipette, a single droplet of urine is deposited onto it. Subsequently, a cover slip is placed over the droplet.4 Examination under bright field microscopy provides better resolution, especially of a stained sample. Dark field microscopy provides lower resolution but allows visualization of objects that are unstained or transparent. It is especially helpful in identification of lipids and crystals as it gives a bright image against a dark background. Phase contrast microscopy is a valuable technique for observing transparent structures such as hyaline casts and particles with a low refractive index. It improves the visibility of the structure’s outline and is especially beneficial for visualizing dysmorphic red blood cells (RBCs).5 Polarized light is useful for identification of lipids, crystals [Table 3],6 and contaminants such as starch and synthetic fibers. At least a minimum of 10 fields (optimally 20) are examined under both low (100×) and high (400×) magnification. The sediment is observed without the use of staining or can be treated with Sternheimer Malbin (SM) stain to differentiate nucleated cells from other structures. Sudan stain can be used for the lipids. The utilization of Wright/Hansel stain for urine eosinophils is no longer recommended, as the presence of urine eosinophils is not considered specific or sensitive enough to diagnose AIN.7
Cells | Identification | Remarks |
---|---|---|
RBCs |
Smooth, biconcave discs, 6–8 μm in diameter, no nucleus In hypertonic urine, RBCs shrink and become crenated In hypotonic urine, RBCs swell and lose their hemoglobin, forming ghost cells |
Normal- 0–3/hpf or 3–12/μl of urine Isomorphic RBCs à nonglomerular or urological Dysmorphic RBCs à glomerular hematuria |
WBCs |
Small, regularly round cells with granular cytoplasm with a size of 10 μm with lobed or segmented nuclei (neutrophils) In hypotonic urine, cells swell with Brownian motion of nuclei and cytoplasmic organelles called as the glitter cells |
Normal- 0–8/hpf or 10/μl of urine Neutrophils are the predominant type of WBCs seen in urine |
RTEC |
Round to oval or rectangular, large central or eccentric nucleus, granular cytoplasm with a size of 14 μm Nucleus cytoplasmic ratio- 1:1 |
Normal- 0–1/hpf Represents damage to tubular epithelium |
Transitional (urothelial) cell |
Round or ovoid cell; round, centrally placed nucleus with an average size of 30 μm Nucleus to cytoplasmic ratio- 1:2 |
Normal- 0–1/hpf Represents the cells lining calyces, renal pelvis, ureter, bladder, proximal urethra (in males) |
Squamous cell | Large cells of rectangular or polygonal shape with small nuclei and abundant cytoplasm with an average size of 55 μm. Nucleus to cytoplasmic ratio- 1:5 | Represents contamination of the urine sample |
hpf: high-power field; RBC: red blood cell; RTEC: renal tubular epithelial cell; WBC: white blood cell.
Casts | Identification | Remarks |
---|---|---|
Acellular casts | ||
Hyaline casts | Colorless fibrillary matrix composed of Tamm–Horsfall protein, low refractile index | Increased numbers with strenuous exercise, dehydration, fever |
Granular casts | Granules can be fine or coarse. Derived from cellular degeneration | Distinguishing finding in acute tubular necrosis, especially if seen with RTEC casts |
Waxy casts | Homogenous, broad, waxy-appearing cast with high refractile index. Frequently cracked or indented edges | Seen in patients with renal failure, both acute and chronic |
Fatty casts |
Contains fat droplets, oval fat body, or cholesterol crystals within the cast matrix Cholesterol globules form Maltese cross under polarized microscopy |
Seen in nephrotic range proteinuria |
Cellular casts | ||
RBC casts | RBCs within the matrix | Distinguishing feature of nephritic syndrome, rarely may also be found with AIN |
WBC casts | WBCs in the matrix | Can be seen in AIN, nephritic syndrome, pyelonephritis |
RTEC casts | RTEC in the matrix | Seen in acute tubular necrosis, AIN |
Others | ||
Pigmented casts |
Hemoglobin casts Myoglobin casts Bilirubin casts |
Associated with RBC casts Seen in rhabdomyolysis Seen in liver failure |
Bacterial casts | Bacteria within matrix | Bacterial pyelonephritis |
Crystal casts | Crystals within cast | Seen with renal calculi formation or drug precipitation |
RBC: red blood cell; RTEC: renal tubular epithelial cell; WBC: white blood cell; AIN: acute interstitial nephritis.
Crystal | pH | Birefringence | Appearance |
---|---|---|---|
Common crystals | |||
Uric acid | ≤5.8 | + | Pleomorphic, diamond, or rhombic; can form layers or rosettes |
Amorphous urates | ≤5.8 | + | Irregular granules |
Calcium oxalate | 5.4-6.7 | mono- | Dihydrate- envelope form |
Calcium phosphate | ≥7.0 | -hydrate) | Monohydrate- dumb bell shaped |
Triple phosphate | ≥7.0 | + | Prism, star-like particles or needles of varying sizes |
Amorphous phosphate | ≥7.0 | + | Coffin lid appearance |
Pathological crystals | - | ||
Cholesterol | 5.4–6.7 | - | Flat, rectangular plates with notched corners |
Cystine | Variable | - | Hexagonal plates, often layered |
2, 8-Dihydroxyadenine | ≤5.8 | + | Spherical brownish crystals with radial striations from the center |
Leucine | ≤5.8 | + | Spheres with concentric circles |
Tyrosine | ≤5.8 | + | Fine delicate needles in clusters |
Due to drugsa | |||
Sulfadiazine | ≤5.8 | + | Shocks of wheat or shells with radial striations |
Acyclovir | ≤5.8 | + | Needles with sharp or blunt ends |
Indinavir | ≤5.8 | + | Irregular plates- crosses/stars like |
Ciprofloxacin | ≤5.8 | + | Needles- stars/fan like |
Amoxicillin | ≤5.8 | + | Shocks of wheat or broom brush like |
Urine sediment examination by different etiologies of AKI
Prerenal AKI
In a study by Mehta et al.,2 prerenal AKI accounted for 17% of AKI cases among hospitalized patients. The urinary sediment typically appears unremarkable or shows the presence of hyaline casts4 in prerenal AKI.
Acute tubular necrosis
In cases of ATN, shedding of tubular epithelium leads to the appearance of renal tubular epithelial cells (RTECs), RTEC casts, and granular casts in the urine sediment.8 Dense and brownish/burnt amber granular casts are known as muddy brown granular casts (MBGC). MBGC have 100% specificity and 100% positive predictive value (PPV) for predicting ATN on biopsy.9 Several scoring systems for urine microscopy have been developed based on the quantification of RTECs, RTEC casts, and granular casts [Table 4]. These scoring systems have excellent specificity for AKI and also correlate well with severity of AKI. The AKI cast scoring index created by Perazella et al.10 showed a PPV of 100% and a negative predictive index (NPV) of 42% for the diagnosis of ATN in hospitalized patients with AKI, when the score was ≥2 (i.e. presence of at least one granular cast or RTECs). These scoring systems are also used to predict a more severe course of AKI. A greater urine sediment score was linked to a higher Acute Kidney Injury Network (AKIN) stage of AKI, and individuals with a urine sediment score of ≥3 had a relative risk of 7.3 for experiencing worsening AKI compared to those with a score of 0.11 According to a study conducted by Chawla et al.,12 patients with a higher cast scoring index had poorer renal recovery compared to those with a lower index (2.55 ± 0.9 vs 1.7 ± 0.79, P = 0.04). The study also showed that the cast scoring index is useful in predicting the nonrenal recovery. Bagshaw et al.13 also concluded that elevated urinary microscopy scores were linked to increased adjustive odds of worsening AKI, and they correlated with initiation of RRT and mortality.
Study | Scoring system |
---|---|
Chawla et al. (2008)12 |
Grade 1: no casts or RTEC Grade 2: at least one cast or RTEC, but <10% of lpf Grade 3: many casts or RTECs (10%-90% of lpf) Grade 4: sheet of MBGC and RTEC in >90% of lpf |
Perazella et al. (2010)10 |
0 points: no casts or RTEC seen 1 point each: one to five casts/lpf or one to five RTECs/hpf 2 points each: ≥6 casts/lpf or ≥6 RTECs/hpf |
Bagshaw et al. (2011)13 |
0 points: no casts or RTEC seen 1 point: one cast/lpf or one RTEC/hpf seen 2 points each: two to four casts/lpf or two to four RTECs/hpf 3 points each: ≥5 casts/lpf or ≥5 RTECs/hpf |
hpf: high-power field; lpf: low-power field; MBGC: muddy brown granular casts; RTEC: renal tubular epithelial cell.
The presence of MBGC was associated with an increased risk of experiencing a ≥50% increase in serum creatinine from baseline at discharge (acute kidney disease).9 The drawbacks of urinary microscopy scoring system include limited validation of these scores in large cohorts and the timing of the urinary sediment assessment after the development of AKI, which may affect the predictive value. Varghese et al. demonstrated that 20%–24% of patients converted from prerenal AKI to ATN based on the urinary microscopy scoring system, only after a repeat urine sediment analysis was performed ≥2 and ≥4 days of the initial analysis serially. Hence, a serial urine sediment analysis is recommended to improve the yield in patients with nonrecovering AKI of unclear etiology.14
Acute interstitial nephritis
AIN encompasses a heterogeneous group of disorders with myriad causes like drug hypersensitivity (e.g. antibiotics, nonsteroidal anti-inflammatory drugs [NSAIDs], anticancer drugs), immunological disorders (e.g. systemic lupus erythematosus, Sjogren’s syndrome), infections (bacterial, viral), and idiopathic. AIN is histologically characterized by edema and leukocytic infiltration of the interstitium, and this reflects in the characteristic urine sediment findings of AIN: white blood cells (WBC), RBCs, and WBC casts. Sterile pyuria is seen in 60%–80% of cases of AIN and is more common in antibiotic-induced AIN. However, lack of pyuria does not rule out the possibility of AIN. Eosinophiluria, earlier a sine-qua-non of AIN, is no longer considered sensitive/specific for AIN, as it is also seen in other pathologies like glomerulonephritis, chronic pyelonephritis, prostatitis, renal atheroembolism, and urinary schistosomiasis.15 RBCs are present in a mean of 50% of cases, making it a nonspecific and insensitive finding for AIN. AIN is also associated with tubulitis and tubular injury, hence RTEC and granular casts are also seen in 86% of cases. RBC casts are generally considered to be associated with glomerular pathology; however, a study conducted by Fogazzi et al.16 revealed presence of RBC casts in 29% of biopsy-proven AIN cases. They proposed that disruption of interstitial blood vessels in AIN could lead to extravasation of RBCs into the tubular lumen, where they mix with uromodulin to form RBC casts. The same study also found WBC casts in only 14% of biopsy-proven AIN, making WBC cast an insensitive finding for diagnosing AIN. Also important to note, 20% of patients with AIN may have a bland urine sediment.
Nephritic syndrome
Nephritic syndrome is characterized by hypertension, proteinuria, active urine sediment, and low glomerular filtration rate. Histologically, acute nephritic syndrome is identified by the presence of intracapillary proliferation, which may or may not be accompanied by the influx of polymorphs or mononuclear cells. In addition, it can manifest as crescent formation (also known as extracapillary proliferation) or glomerular fibrinoid necrosis. This is reflected in the urine sediment analysis as erythrocyturia, leukocyturia, shedding of RTECs, RBC casts, and mixed cellular casts.7 It was Fairley and Birch who first suggested dysmorphic RBCs to be a hallmark of glomerular bleeding.17 Dual injury, an initial mechanical damage caused by the passage of RBCs through the glomerular basement membrane followed by osmotic injury during passage through hypotonic tubular fluid, is responsible for dysmorphism of RBCs.18 Dysmorphic RBCs exhibit irregular central pallor with uneven edges or a distinct target-like appearance. In certain cases, these dysmorphic RBCs may also display small blebs or protrusions. On the other hand, isomorphic RBCs possess a biconcave shape with a central pallor that accounts for approximately half of the RBC diameter. The central pallor displays smooth edges. In urine, these RBCs have the ability to either swell into spherical shapes or shrink into spiked disc. Chu-Su et al.19 documented 28 types of isomorphic and dysmorphic RBCs based on different morphologies. Acanthocytes or G1 cells with membrane protrusions or blebs and doughnut-shaped deformity (loss of cytoplasmic color due to reduced hemoglobin content) are considered to be the most characteristic dysmorphic RBCs seen in glomerular bleeding. The importance of phase contrast microscopy in identifying these dysmorphic RBCs cannot be overemphasized. However, with lack of access to phase contrast microscopy, bright field microscopy with lowering of condenser and SM staining can be used to improve the yield. There is a lack of clear and consistent criteria to definitively distinguish hematuria as either glomerular or nonglomerular in origin. The literature reports varying clinical cut-offs for significant dysmorphic RBCs, ranging from 10% to 90%, based on the study design and the selected population.20-22 Hamadah et al.23 conducted a study using a dysmorphic RBC threshold of ≥25% and found that it yielded a sensitivity of 20.4% and a specificity of 96.3% for glomerular diseases. In addition, PPV was determined to be 94.6% in this context. Based on these various studies, presence of ≥40% dysmorphic RBCs and/or ≥5% acanthocytes and/or ≥1 RBC cast/50 low-power fields (lpf) is a reasonable cut-off to define glomerular hematuria.7 Acanthocytes and dysmorphic RBCs serve as relatively specific markers for glomerular damage; however, they exhibit limited sensitivity as isomorphic RBCs are often seen in glomerulonephritis. Isomorphic RBCs may also appear in different nonglomerular situations such as AIN and renal cell carcinoma, as well as in conditions outside the kidneys like nephrolithiasis, urologic cancers, urinary tract infections (UTIs), and excessive anticoagulation. Glomerular injury per se without any evidence of UTI may have pyuria and WBC casts. Their presence in association with dysmorphic RBCs/RBC cast is more suggestive of a proliferative glomerulonephritis rather than a nonproliferative glomerulonephritis.24 Coexistence of tubular damage, in association with acute nephritic syndrome explains the presence of RTECs and RTEC casts in the urine sediment. Besides aiding in the diagnosis, the presence of hematuria, dysmorphic RBCs, and RBC casts is also valuable in assessing the response to treatment and predicting the probability of relapse, particularly in patients with lupus nephritis and pauci-immune vasculitis.25,26
Nephrotic syndrome
Nephrotic range proteinuria (≥3.5 g/day), hypoalbuminemia (<2.5 g/dl), and edema are considered the characteristic triad of nephrotic syndrome,27 which reflects in urine sediment analysis as lipiduria and fatty casts.
Nephrotic syndrome is associated with hyperlipidemia, and lipiduria is thought to be due to ultrafiltration of these lipid fragments, majorly high-density lipoprotein (HDL) particles because of their relatively small size, across the hyperpermeable glomerular basement membrane. Inside the tubules, proximal tubular cells partially reabsorb lipids and transport them to lysosomes for hydrolysis. These lipid-filled tubular cells slough and become oval fat bodies. Within the urinary sediment, lipids can manifest in different forms, such as free-floating fat droplets, or within renal tubular cells or macrophages (oval fat bodies), or within tubular cast (fatty casts), or as cholesterol crystals. Free lipid droplets appear as translucent round particles, either isolated or in aggregates. Under the polarized light, cholesterol and its esters (not other lipids) produce the typical appearance of “Maltese crosses” with symmetrical arms. Lipid droplets could be mistaken for isomorphic RBCs, yeast, round calcium oxalate crystals, or impurities like starch particles (Maltese crosses, with asymmetrical arms under polarized light). Cholesterol crystals are thin, colorless, and transparent plates exhibiting distinct edges and displaying negative birefringence, formed in vitro, in refrigerated urine samples.7 The lipid in the oval fat body and casts can be composed of either cholesterol or neutral fat (triglycerides). Sudan III or IV stains both cholesterol and triglycerides, whereas oil red stains only triglycerides. In the absence of staining, combination of phase contrast microscopy and polarized microscopy is ideal for identification of lipids.28 Lipiduria is traditionally a marker of nephrotic syndrome, although it may not be invariably present. In a study by Ravigneaux et al.,29 lipiduria was seen in 62.8% of cases with nephrotic syndrome under phase contrast microscopy. They concluded that lipiduria is not predictive of the severity of renal histologic findings. Of note, lipiduria may be seen in non-nephrotic conditions like autosomal dominant polycystic kidney disease (ADPKD),30 Fabry’s disease,31 chyluria,32 lipid emulsion therapy,33 prostatitis, and oil contamination of the urine specimen.5
Crystalluria
Crystals are structures that result from the solidification of urinary solutes in urine. These urinary solutes can consist of a single element, a compound, or a combination of substances, and they are organized in a consistent, repetitive pattern within the crystalline structure. The crystal formation depends on multiple factors like concentration of solute in the urine, the urine pH, and flow of urine through the tubules.5 For ease of understanding, the crystals can be classified into common crystals, pathological crystals, and crystals due to drugs.34,35 They can be differentiated on the basis of their structure, urinary pH at which they precipitate, and birefringence under polarizing microscopy, as highlighted in Tables 3 and 5.
Crystals | Associated conditions |
---|---|
Common crystals | |
Uric acid |
Normal subjects, refrigerated urine sample, hyperuricemia secondary to tumor lysis syndrome34 |
Amorphous urates | Normal subjects, refrigerated sample |
Calcium oxalate | Normal subjects, primary and secondary hyperoxaluria, ethylene glycol ingestion |
Calcium phosphate | Normal subjects and stone formers |
Triple phosphate | Normal subjects. Bacterial infections caused by urea-splitting organisms such as Ureoplasma ureolyticum and Corynebacterium ureolyticum35 |
Amorphous phosphates | Normal subjects, refrigerated sample |
Pathological crystals | |
Cholesterol | Nephrotic syndrome, chyluria |
Cysteine | Cystinuria – autosomal recessive defect in dibasic amino acid transporter |
2,8-Dihydroxyadenine | APRT enzyme deficiency |
Leucine | Liver failure |
Tyrosine | Inherited disorders of tyrosine metabolism, liver failure |
APRT: adenine phosphoribosyl transferase.
Automated systems for urine sediment analysis
Automated analyzers enable the rapid assessment of a large quantity of uncentrifuged samples within a brief timeframe. Frequently used autoanalyzers are IRIS iQ200, Sysmex UF-1000i, Cobas u701, and Sedimax.4 Automated intelligent microscopy (AIM) encompasses fluids, electro-optics, and image processing. The commonly utilized iQ200 system employs laminar flow technology that aspirates 1 ml of unspun urine and passes 2 μl through a flow chamber, which lies in the object plane of a microscope. In this plane, using stroboscopic illumination, hundreds of images are captured by the charge coupling device (CCD) camera. The camera is equipped with an advanced auto particle recognition software, which uses size, shape, contrast, and texture to identify 12 particle categories of the urine sediment, including cells, casts, crystals, and microorganisms. Due to its multistep nature, manual urine microscopy can be a labor-intensive and time-consuming process involving steps such as centrifugation, slide preparation, and thorough examination of the urine specimen. Lack of methodological standardization and poor interobserver reliability also pose hurdles for proper utilization of manual analysis. Automated urinalysis systems are, therefore, considered time-saving, cost-effective, and standardized systems.
However, in the setting of AKI and in patients with kidney diseases, automated systems have limited effectiveness due to their inadequacy to identify sediment particles such as nonhyaline casts, nonsquamous epithelial cells, dysmorphic RBCs, lipids, and crystals in likely pathological samples (partly secondary to the use of an unspun urine).7 In a limited study conducted at a single center, which included 25 patients diagnosed with ATN, the iQ200 system detected the presence of granular casts in 24% of the samples. Conversely, when the samples were examined by a nephrologist using manual microscopy, granular casts were identified in 72% of the samples (P < 0.001).36 In a different study, automated systems exhibited a strong correlation for erythrocytes (r = 0.87, P = 0.001) and leukocytes (r = 0.92, P = 0.001). However, there wasno notable correlation for pathological nonepithelial cells (r = 0.16, P = 0.049), and the correlation for crystals was very weak (r = 0.46, P = 0.001).37
Urine sediment analysis versus urinary biomarkers in AKI
The last decade has seen a growing interest in developing novel urinary biomarkers to detect AKI early and accurately, as a means to improve the outcomes. The US Food and Drug Administration (FDA)-approved tissue inhibitor of metalloproteinase-2/insulin-like growth factor-binding protein 7 (TIMP-2* IGFBP7) has an area under the receiver operating characteristic curve (AUC) of 0.80 for early identification of AKI and is significantly superior to all previously reported markers of AKI (Kidney injury molecue-1 (KIM-1), Urinary neutrophil gelatinase-associated lipocalin (NGAL), Interleukin-18 (IL-18)).38 Apart from diagnostic potential, the urinary biomarkers have utility in predicting the progression of AKI and determining necessity for dialysis once AKI is established. Higher urinary IL-18 and NGAL levels after AKI sets in identify individuals with high risk of AKI progression and worse patient outcomes.39 Elevated levels of urinary Chemokine (C-C motif) ligand 14 (CCL-14) in patients with stage 2/3 AKI following cardiac surgery demonstrated a strong predictive value for the requirement of renal replacement therapy (RRT) within the next 7 days, with an AUC of 0.91.40 However, these biomarkers have their own set of limitations. None of the biomarkers reported are completely specific to AKI. They can also be elevated in other conditions such as sepsis, chronic kidney disease (CKD), and UTIs and do not have specific cut-off values that can be universally applied.41,42 They perform poorly in patients with preexisting CKD and other comorbidities.43 Currently, there is inadequate evidence to support the notion that early detection of AKI through the use of biomarkers effectively prevents the progression of AKI or proves to be cost-effective.44
Studies comparing the urine sediment analysis and urinary biomarkers in AKI have found a good correlation between higher urine microscopy scores and elevated urinary NGAL levels (P = 0.0003).13 Similar predictive value was also found between urine microscopy and urinary KIM-1, IL-18, and urinary NGAL.45 In terms of reliability in diagnosing AKI, urinary NGAL is more sensitive (sensitivity- 65%, specificity- 65%, AUC- 0.70) and urinalysis with microscopy is more specific (specificity- 91%, sensitivity- 22%, AUC- 0.57) for diagnosing AKI. Like the urinary biomarkers, urine sediment analysis also helps in prognostication. In a study by Perazella et al.,11 higher sediment score was found to be associated with an increasing adjusted relative risk of worsening AKI (adjusted relative risk: 7.3, 95% confidence interval: 4.5 to 9.7 for worsening with a score of ≥ 3 vs. a score of 0).
Quality control
The role of a good internal quality control and external quality assessment to improve the quality and reliability of urine sediment examination cannot be overemphasized. Standardizing the method of preparing the urine sediment is the first step to achieve this. Avoiding delays in investigation after micturition, standardization of centrifugation time and speed, avoiding losses during centrifugation, use of suction rather than decanting to remove the supernatant, gentle resuspension of the pellet to avoid lysis of any casts, and adequate review of the full coverslip area are some of the steps that need strict adherence to improve the yield.3 Being cognizant of the potential for errors in these stages should serve as a guiding principle when developing the most precise protocol for routine sediment analysis. The identification of clinically significant urine particles should be internally reviewed and externally evaluated (External Quality Assessment schemes). In a small-scale study conducted at a single center involving 26 patients, the accuracy of urine sediment interpretation was compared between laboratory personnel and a nephrologist. The findings revealed that the nephrologist, utilizing manual microscopy, detected a higher number of RTECs, granular casts, and dysmorphic RBCs compared to the interpretations made by laboratory personnel. Even when blinded to clinical history, the nephrologist conducting urinary microscopy achieved accurate diagnoses in over 90% of cases. In contrast, when a second nephrologist relied on automated urinalysis and laboratory-based microscopy report, the correct diagnosis was made only 19% of the time.46 In a study conducted by Palsson et al.,47 the consistency among nephrologists in identifying various urine sediment findings exhibited mostly moderate to substantial interobserver reliability, but the level of agreement varied substantially. On the other hand, some findings like WBC casts and RTECs showed only fair agreement among the observers. The above studies highlight the importance of manual urine sediment examination and the crucial role of nephrologists in the analysis, advocating the need for sufficient training in this vital cost-effective procedure.
Limitations of urine microscopy
Although it is cost-effective and capable of offering valuable diagnostic insights, urine microscopy analysis still comes with its own array of constraints. It is essential to stress the importance of standardizing procedures, conducting prompt examinations, following sample collection to prevent artifacts and crystallization and addressing interobserver differences, which necessitates the significance of incorporating proper training into the curriculum. In addition, the lack of universal access to phase contrast microscopy and special stains poses obstacles to the precise identification of certain sediment particles. Although urine sediment scores have been used to predict the severity and prognosis of AKI, there is limited validation of these scores in larger cohorts.48 On occasion, urine sediment may appear unremarkable even when various underlying kidney conditions, such as AIN, proliferative lupus glomerulonephritis, acute tubular injury, and thrombotic microangiopathy, are present.4 Furthermore, the cells and crystals observed in urine may not necessarily indicate the underlying cause of the disease. Notable examples include uric acid, calcium oxalate, and drug-related crystals found in the urine of asymptomatic individuals. Hence, the importance of a physician’s judgment based on the clinical scenario and pretest probability for a likely diagnosis cannot be overemphasized.
Conclusion
Once an inclusive part of nephrology curriculum, the practice of urine microscopy involving the examination of urine sediment is gradually waning and becoming a dying art for nephrologists. But with the ease of performing, cost-effectiveness, and the ability to make diagnostic decisions leading to timely intervention, especially in the context of AKI, urine examination is here to stay. The importance of this art cannot be overemphasized in the current scenario, with the infiltration of nephrologists to second- and third-tier cities which lack trained and equipped central laboratory services at their disposal. So, it is time for nephrologists to go back to spinning the urine, to add a new dimension to the comprehensive patient care that every patient deserves.
Conflicts of interest
There are no conflicts of interest.
References
- A History of urine microscopy. Clin Chem Lab Med. 2015;53:s1453-64.
- [CrossRef] [PubMed] [Google Scholar]
- Spectrum of acute renal failure in the intensive care unit: The PICARD experience. Kidney Int. 2004;66:1613-21.
- [CrossRef] [PubMed] [Google Scholar]
- European urinalysis guidelines. Scand J Clin Lab Investig. 2000;60(Supp 231):1-96.
- [CrossRef] [PubMed] [Google Scholar]
- Urine sediment examination in the diagnosis and management of kidney disease: Core curriculum 2019. Am J Kidney Dis. 2019;73:258-72.
- [CrossRef] [PubMed] [Google Scholar]
- Fundamental of urine and body fluid analysis. China: Elsevier; 2018.
- The urinary sediment. An integrated view. Penerbit Buku Kompas; 2010.
- Urinary eosinophils in AIN: Farewell to an old biomarker? Clinical Journal of the American Society of Nephrology. 2013. ;8:1841-3.
- [Google Scholar]
- Concomitant identification of muddy brown granular casts and low fractional excretion of urinary sodium in AKI. Kidney360. 2022;3:627-35.
- [CrossRef] [PubMed] [PubMed Central] [Google Scholar]
- Diagnostic value of urine microscopy for differential diagnosis of acute kidney injury in hospitalized patients. Clin J Am Soc Nephrol. 2008;3:1615-9.
- [CrossRef] [PubMed] [PubMed Central] [Google Scholar]
- Urine microscopy is associated with severity and worsening of acute kidney injury in hospitalized patients. Clin J Am Soc Nephrol. 2010;5:402-8.
- [CrossRef] [PubMed] [PubMed Central] [Google Scholar]
- Urinary sediment cast scoring index for acute kidney injury: A pilot study. Nephron Clin Pract. 2008;110:c145-50.
- [CrossRef] [PubMed] [PubMed Central] [Google Scholar]
- A prospective evaluation of urine microscopy in septic and non-septic acute kidney injury. Nephrol Dial Transplant. 2012;27:582-8.
- [CrossRef] [PubMed] [Google Scholar]
- Diagnostic utility of serial microscopic examination of the urinary sediment in acute kidney injury. Kidney360. 2021;2:182.
- [CrossRef] [PubMed] [PubMed Central] [Google Scholar]
- Utility of urine eosinophils in the diagnosis of acute interstitial nephritis. Clin J Am Soc Nephrol. 2013;8:1857-62.
- [CrossRef] [PubMed] [PubMed Central] [Google Scholar]
- Urinary sediment findings in acute interstitial nephritis. Am J Kidney Dis. 2012;60:330-2.
- [CrossRef] [PubMed] [Google Scholar]
- Hematuria: A simple method for identifying glomerular bleeding. Kidney Int. 1982;21:105-8.
- [CrossRef] [PubMed] [Google Scholar]
- What makes red cells dysmorphic in glomerular haematuria? Pediatr Nephrol. 1992;6:424-7.
- [CrossRef] [PubMed] [Google Scholar]
- Enhancing the detection of dysmorphic red blood cells and renal tubular epithelial cells with a modified urinalysis protocol. Sci Rep. 2017;7:40521.
- [CrossRef] [PubMed] [PubMed Central] [Google Scholar]
- Diagnostic value of urinary dysmorphic erythrocytes in clinical practice. Nephron Clin Pract. 2010;115:c203-12.
- [CrossRef] [PubMed] [Google Scholar]
- Urine erythrocyte morphology in patients with microscopic haematuria caused by a glomerulopathy. Pediatr Nephrol. 2008;23:1093-100.
- [CrossRef] [PubMed] [Google Scholar]
- The Value of urinary red cell shape in the diagnosis of glomerular and post-glomerular haematuria. A meta-analysis. Postgrad Med J. 1992;68:648-54.
- [Google Scholar]
- Urinalysis for the diagnosis of glomerulonephritis: Role of dysmorphic red blood cells. Nephrol Dial Transplant. 2018;33:1397-403.
- [CrossRef] [PubMed] [Google Scholar]
- Urinary sediment features in proliferative and non-proliferative glomerular diseases. J Nephrol. 2005;18:703-10.
- [PubMed] [Google Scholar]
- Relationship between appearance of urinary red blood cell/ white blood cell casts and the onset of renal relapse in systemic lupus erythematosus. Am J Kidney Dis. 1995;26:432-8.
- [CrossRef] [PubMed] [Google Scholar]
- Level of red blood cells in the urinary sediment reflects the degree of renal activity in Wegener’s granulomatosis. Clin Nephrol. 1998;50:284-8.
- [PubMed] [Google Scholar]
- Minimal change disease. Clin J Am Soc Nephrol. 2017;12:332-45.
- [CrossRef] [PubMed] [PubMed Central] [Google Scholar]
- Color atlas of the urinary sediment: An illustrated field guide based on proficiency testing. Northfield: College of American Pathologists; 2019.
- Significance of cytolipiduria during a nephrotic syndrome. Nephrologie. 1991;12:12-6.
- [PubMed] [Google Scholar]
- Urinary lipid bodies in polycystic kidney disease. Am J Kidney Dis. 1985;5:49-53.
- [CrossRef] [PubMed] [Google Scholar]
- Lipiduria After intralipid infusion of a lipid emulsion in a boy with an abdominal trauma. J Parenter Enteral Nutr. 1991;15:572-3.
- [CrossRef] [PubMed] [Google Scholar]
- A rapid method for the diagnosis of acute uric acid nephropathy. Arch Intern Med. 1978;138:612-5.
- [PubMed] [Google Scholar]
- Clinical value of crystalluria and quantitative morphoconstitutional analysis of URINARY calculi. Nephron Physiol. 2004;98:31-6.
- [CrossRef] [PubMed] [Google Scholar]
- Manual urine microscopy versus automated urine analyzer microscopy in patients with acute kidney injury. Lab Med. 2014;45:e152-5.
- [CrossRef] [PubMed] [Google Scholar]
- Performance evaluation and comparison of the fully automated urinalysis analyzers UX-2000 and Cobas 6500. Lab Med. 2016;47:124-33.
- [CrossRef] [PubMed] [Google Scholar]
- TIMP2• IGFBP7 biomarker panel accurately predicts acute kidney injury in high-risk surgical patients. J Trauma Acute Care Surge. 2016;80:243-9.
- [CrossRef] [PubMed] [PubMed Central] [Google Scholar]
- Biomarkers predict progression of acute kidney injury after cardiac surgery. J Am Soc Nephrol. 2012;23:905-14.
- [CrossRef] [PubMed] [PubMed Central] [Google Scholar]
- Comparison of C-C motif chemokine ligand 14 with other biomarkers for adverse kidney events after cardiac surgery. J Thoracic Cardiovasc Surg. 2023;165:199-207.e2.
- [CrossRef] [PubMed] [Google Scholar]
- Neutrophil gelatinase-associated lipocalin as a biomarker of acute kidney injury––Where do we stand today? Nephrol Dial Transplant. 2011;26:762-4.
- [CrossRef] [PubMed] [Google Scholar]
- Evaluation of novel biomarkers of acute kidney injury: The possibilities and limitations. Curr Med Chem. 2016;23:1981-97.
- [CrossRef] [PubMed] [Google Scholar]
- Neutrophil gelatinase-associated lipocalin and acute kidney injury after cardiac surgery: The effect of baseline renal function on diagnostic performance. Clin J Am Soc Nephrol. 2010;5:211-9.
- [CrossRef] [PubMed] [PubMed Central] [Google Scholar]
- Cell-cycle arrest and acute kidney injury: The light and the dark sides. Nephrol dial transplant. 2016;31:16-22.
- [CrossRef] [PubMed] [PubMed Central] [Google Scholar]
- Risk of poor outcomes with novel and traditional biomarkers at clinical AKI diagnosis. Clin J Am Soc Nephrol. 2011;6:2740-9.
- [CrossRef] [PubMed] [PubMed Central] [Google Scholar]
- Comparison and interpretation of urinalysis performed by a nephrologist versus a hospital-based clinical laboratory. Am J Kidney Dis. 2005;46:820-9.
- [CrossRef] [PubMed] [Google Scholar]
- assessment of interobserver reliability of nephrologist examination of urine sediment. JAMA network open. 2020;3:e2013959.
- [CrossRef] [PubMed] [PubMed Central] [Google Scholar]
- Urine sediment exam provides more diagnostic information in AKI than novel urinary biomarkers: Commentary. Kidney360. 2022;3:604-7.
- [CrossRef] [PubMed] [PubMed Central] [Google Scholar]